Research
Development of innovative solid CO2 absorbents
Background and need for research
Atmospheric CO2 concentrations are increasing at an accelerating rate each year (NOAA, USA), casting doubt on the viability of the human race. According to the IEA's roadmap published in 2021, global CO2 emissions should have been slowing down by 2024 and reduced to around 30 billion tons per year (p. 152 of the roadmap), but in reality, it has been reported that the global emission has exceeded the 40 billion ton (40 gigatons) mark in 2023 (Energy Institute, UK, 2024). It has been scientifically proven that the increase in atmospheric CO2 concentration contributes to global warming (Syukuro Manabe, Nobel Prize in Physics 2021) and that the increase in atmospheric CO2 concentration is primarily anthropogenic (IPCC AR6 WG1, Chapters 1 & 3; see also the website of the Royal Society). In fact, the global temperature has been steadily increasing (Copernicus, EU), and in particular from 2023 to 2024, it clearly exceeded the previous global average temperature and set a new record high (Copernicus, EU).
The recent climate changes are causing natural disasters (UNEP), hungers (WFP), and health problems (WHO) on a global scale. From a different perspective, some researchers pointed out fundamental problems with humans as a species, such as the concern that humans may not be able to maintain concentration (and hence intellectual activities may be hindered) if CO2 concentrations exceed 1000 ppm (Satish et al., 2012). In this way, the problem of rising atmospheric concentrations of CO2 not only jeopardizes the future survival of humanity, but also poses an imminent danger to our current lives and the order and well-being of our society.
When considering solutions to this problem, the most important perspective is the "quantity perspective." As mentioned above, humans currently emit approximately 40 billion tons of CO2 annually. Japan emits about 1 billion tons annually (Ministry of the Environment of Japan, April 2022). In particular, Japan's top 10 emission sources emit more than 10 million tons at a single location (Kiko Network, 2017, in Japanese).
In recent years, research on carbon capture and utilization (CCU) has become active. While CCU has a certain significance as a way to respond to economic and social requirements to reduce emissions through carbon pricing or ESG viewpoint, the following three perspectives are considered important. (1) Is the amount of its reuse meaningful compared to CO2 emissions of the above order? (2) How does the CO2 used for reuse come to be separated? (3) Energy input is required for CO2 separation and reactions such as CO2 reduction (and in some cases, the generation of hydrogen to be reacted with CO2), but are these energies available? In other words, if the context is to solve the climate change problem, a quantitative and systematic perspective is needed.
A representative technology that aims for a quantitative solution is the geological sequestration of CO2, known as CCS, which is already in large-scale operation around the world (Global CCS Institute). However, the more we envision the capture of CO2 from large-scale emission sources, the more we are faced with the question of how to do it, and whether the energy and equipment costs for capture are realistic. The current technology is the aqueous amine solution method described next; however, this has its own problems and therefore research is needed to be conducted to solve the problems.
Problems with existing technology and points that require improvement
The current technology is a chemical absorption method that uses an aqueous solution of amine molecules (e.g., primary amines are -NH2), in which CO2 molecules react with the amine molecules in the solution and are captured (Renew. Sustain. Energy Rev., 2022). When the CO2 is desorbed (or the solution is regenerated), thermal energy must be applied to break the chemical bonds formed between the CO2 and the amine molecules. However, the primary problem with the current technology is that it is also necessary to heat the solvent water, which makes up most of the weight of the liquid (sensible heat), and to input the additional large amount of thermal energy to turn the water into vapor (latent heat). Although improvements in absorbent solutions are currently being made (COURSE50, in Japanese), they are mainly based on efforts to reduce the heat of reaction between amine molecules and CO2 molecules, not on reducing the sensible and latent heat of water as a solvent. There is a limit to the approach of lowering the heat of reaction, because the reaction rate and heat of reaction are inversely related (COURSE50, in Japanese). Therefore, lowering the heat of reaction too much will result in an economically unacceptable equipment size due to the need to increase the contact time between the amine solution and flue gas, since liquid is generally a slow diffusion medium for gas molecules.
In addition, aqueous solutions of amines are highly corrosive to metals and harmful to the ecosystem and human health (Renew. Sustain. Energy Rev., 2022), which increase, respectively, the cost for frequent maintenance inspections of equipment and replacement of amine solutions and cost for scrubber facilities to prevent the release of solute amine molecules into the environment. In other words, if we are aiming to lower the heat of reaction, dramatically reduce the energy input required in the regeneration process, and solve environmental problems, we need to stop using aqueous solutions.
Therefore, the necessary improvements are (1) to avoid using water, which causes excess heat capacity (sensible & latent heat) and low gas diffusion coefficient, i.e., to make it solid, (2) to ensure high reaction rate by using porous solids rather than tight solids, and (3) not to use support materials that can cause excess heat capacity and weight. Among them, (2) is necessary to avoid the above-mentioned dilemma that reaction rate and reaction heat are in a trade-off relationship. If gas diffusion and reaction are faster in porous media, the heat of reaction, which consists a part of energy input required in the regeneration process, can be reduced without side effects.
Idea and aim
The idea of this research is to use "Covalent Organic Frameworks (COFs)" explained below, as a porous material that can effectively solve the above-mentioned problems. COFs are crystalline organic porous materials that have attracted much attention recently because they are composed only of light elements (usually C, H, O, and N), metal-free, highly durable because they are constructed with covalently bonds, and have nanoscale pores that are sufficiently larger than CO2 molecules. COFs are, to say, "jungle gyms" consisting of molecular rods, and their pore size and function can be designed by selecting building block molecules. We believe that using COF, specifically if amine adsorption sites can be densely arranged on the COF's skeleton, we can create the ultimate solid CO2 absorbents that are solvent-free, support-free, porous, highly stable, and consisted of only light elements.
Because COFs are organic solids, they must be kept below 200°C for long-term use in the presence of oxygen. This is much lower temperature than the temperatures used in the methods that use tight-lattice inorganic materials; this is because the gas diffusion coefficient (or mass transfer coefficient) of tight-lattice inorganic materials is small and hence such inorganic materials require use of high temperatures to ensure the reaction rate.
Furthermore, the use of relatively low temperatures has principled advantages based on thermodynamics. Simply put, the act of separating gases to lift off the mixing state at a high temperature will in principle requires higher energy input for the gas separation, because a high temperature favors disorder or the state with increased entropy. If one wants to reduce the energy input for CO2 separation, separation and recovery should be performed at as a low temperature as possible, which is rooted in the fundamental principles of science. (Note: There is a limit because too low temperature slows down the rate of molecular diffusion and desorption within the material, but if there is a "porous material with low heat of adsorption," that will be avoided. If a tight-solid or liquid is used, however, the mass transfer coefficient will be lowered, so higher temperatures are needed to ensure the reaction rate.)
We are conducting such research from the design and synthesis of COFs, their characterization, thermodynamic and kinetic evaluations, and system-level performance evaluations, in order to create an innovative solid CO2 separation and capture method that can be deployed on a large scale and in quantities for effective contribution to climate change mitigation. We recently published a paper reporting a new type of COFs with excellent CO2 capture properties (link to the paper); we are currently expanding this innovation to achieve even higher performance and lower costs to realize the industrialization of this technology.
Development of innovative solid electrolytes for solid-state batteries
Background and need for research
Under construction.
Problems with existing technology and points that require improvement
Under construction.
Idea and aim
Under construction.
About covalent organic frameworks (COFs)
Covalent Organic Framework (COF) is a relatively new material genre, first reported in 2005 (2D-COFs) and 2007 (3D-COFs). This is an emerging category of self-assembled organic materials that are constructed by covalently and regularly polycondensing the building block molecules to form a periodic and porous framework. COF formed with covalent bonds can also be viewed as a large single molecule. Because no substance is finer than a molecule, this is the finest "jungle gym" that can be created. The chemical function and the length of the jungle gym rods (or the pore size) can be designed by choosing building block molecules, allowing for predictable function. Since they are basically composed of only light elements, they are metal-free, lightweight, easy to dispose of, and environmentally friendly. Covalent bonds contribute to the high thermal and chemical stability of the material. Because of these advantageous features, COFs have been proposed for a variety of innovative applications.
We started working with COF in 2018, and have so far grown high-quality crystals by controlling nucleation (Wang et al., 2021), created composite heat storage materials (Murakami et al., 2023), and achieved structure diversification through active control of the skeletal structure (Wang et al., 2024). We believe that the two aforementioned issues can be effectively solved by utilizing the features of COFs and are currently conducting these research projects.
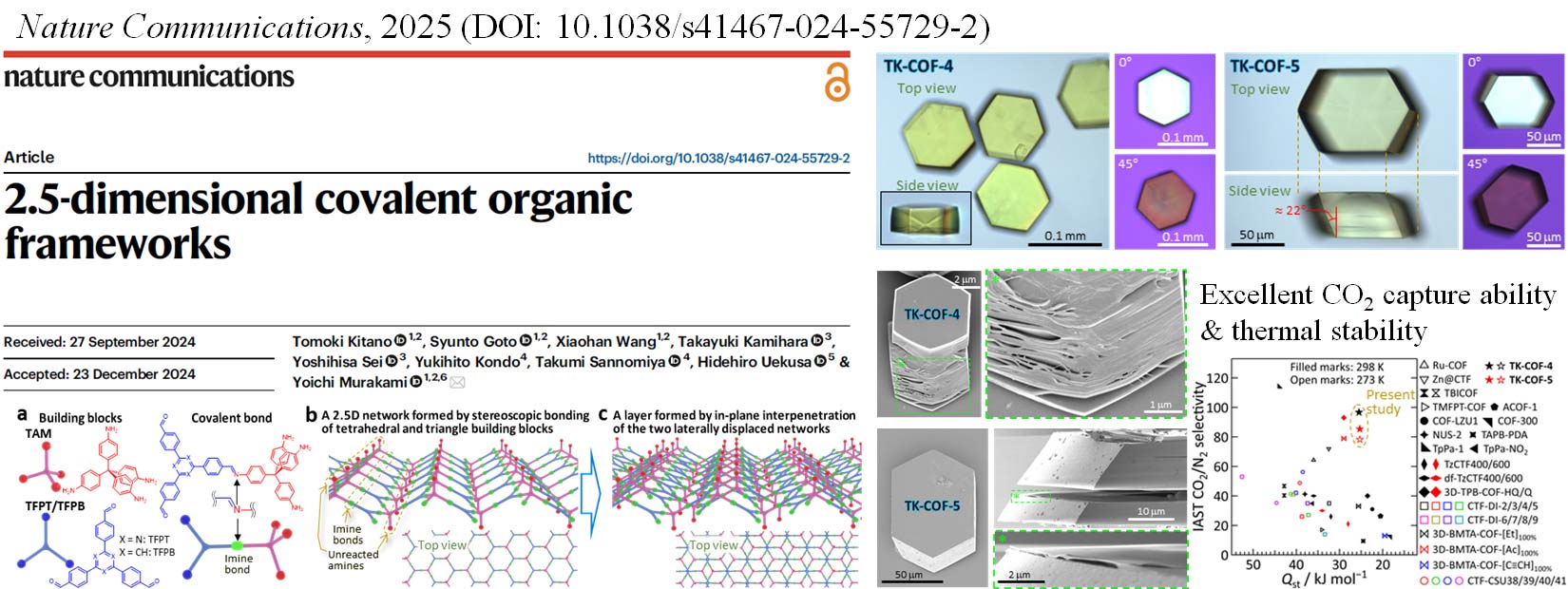
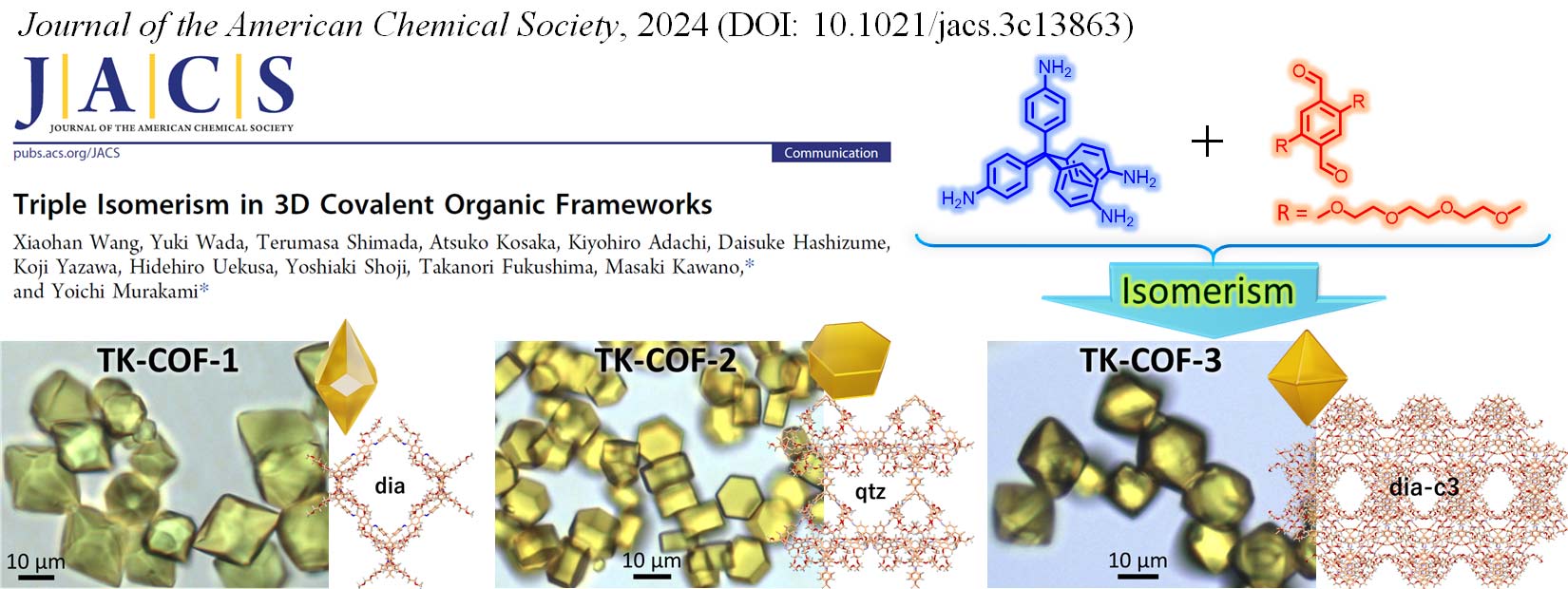
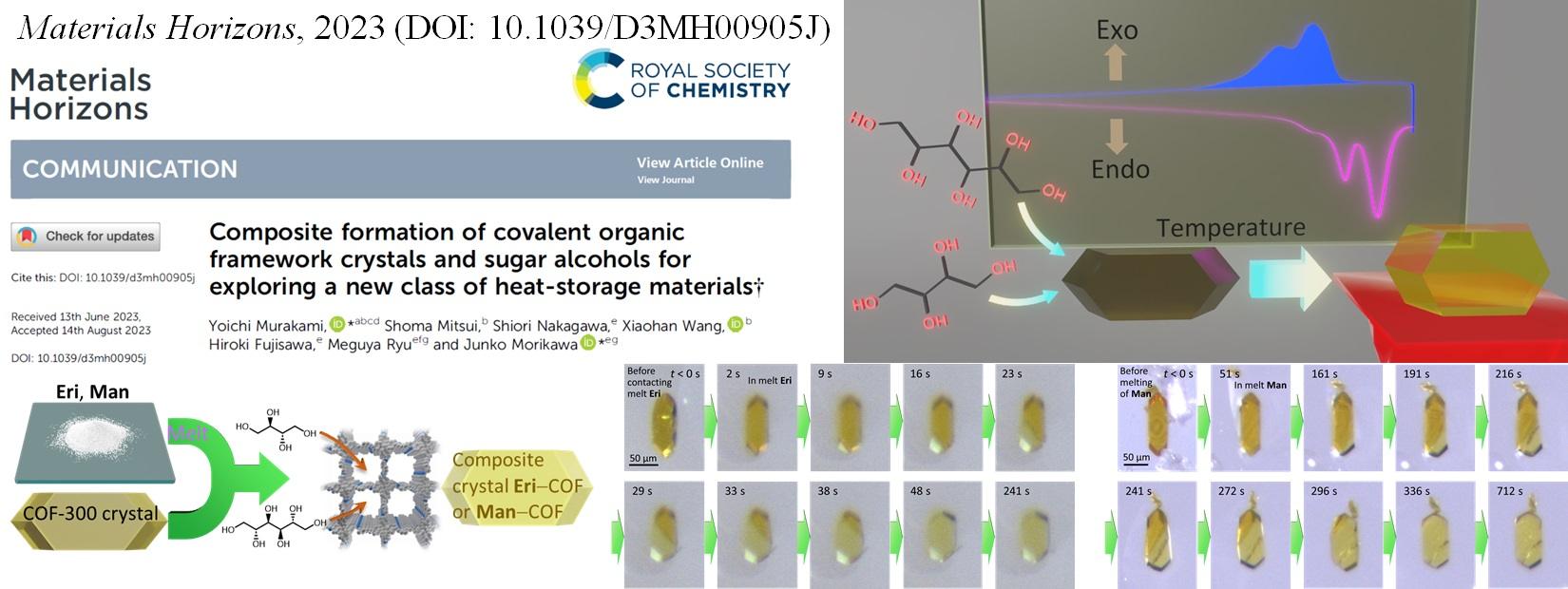
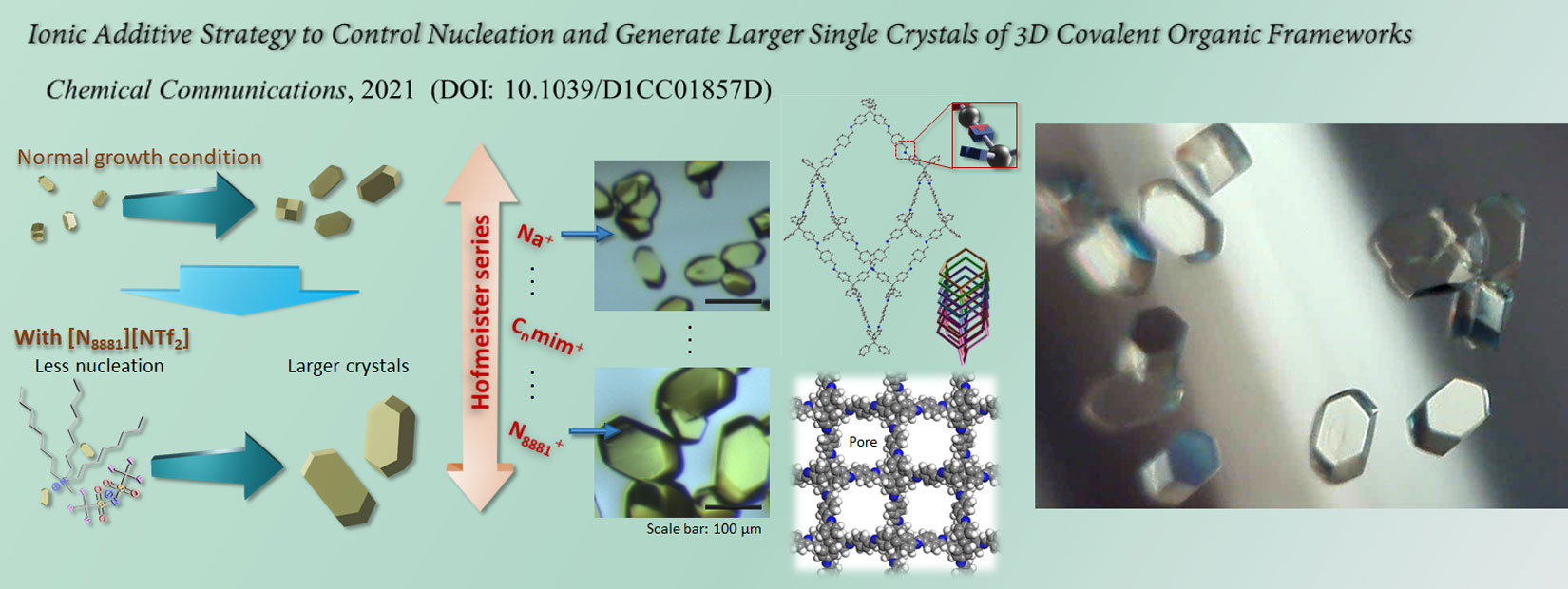
Electrochemical power generation during forced convection cooling to utilize waste heat
In our present civilization, forced convection cooling is important in technologies from microprocessors in data centers, which now consume 1%–2% of global electricity generation, to heat engines including turbines and automobile engines. These technologies require active cooling to avoid thermal failure (for microprocessors) and maximize fuel-to-work conversion efficiency (heat engines). Here, “active cooling” means the prompt removal of a large quantity of thermal energy in the heat source by a working fluid under a large temperature difference. However, such active cooling leads to prompt destruction of the exergy of thermal energy, which is the free-energy component of the thermal energy. This issue has remained unaddressed despite the widespread use of forced convection cooling. If this issue is resolved, it could strongly influence the development of future energy technologies.
The purpose of this research project is to partly recover presently lost exergy in such cooling situations. In particular, we aim at an integration of thermoelectrochemical conversion, which has mostly been studied for stationary conditions using a liquid electrolyte in a closed cell, into forced convection cooling using an electrolyte as the coolant. To fulfill this purpose, we have designed a test cell, in which an electrolyte liquid is forced through a channel formed between two parallel electrodes and the hot-side electrode simulates an object that needs to be cooled.
So far, we have conducted investigations using ionic liquids (room-temperature molten salts composed entirely of ions) as the solvent of redox couple; this solution is forced through the cell as a coolant. The virtual non-flammability and non-volatility of ionic liquids are suitable for wide-ranging heat sources where safety is concerned and situations including uses in space where environment is vacuum, respectively. From our experiments and computational simulations, several findings regarding the fundamental properties of this kind of forced-flow thermocell have been acquired.
However, to bring this concept to real application, further improvements are required. The required improvements include the design optimization of the geometry of liquid flowing channel in the cell, establishment of strategies for scale-up of the cell dimensions, and optimizations of both electrode materials and redox couple chemicals used in the cell.
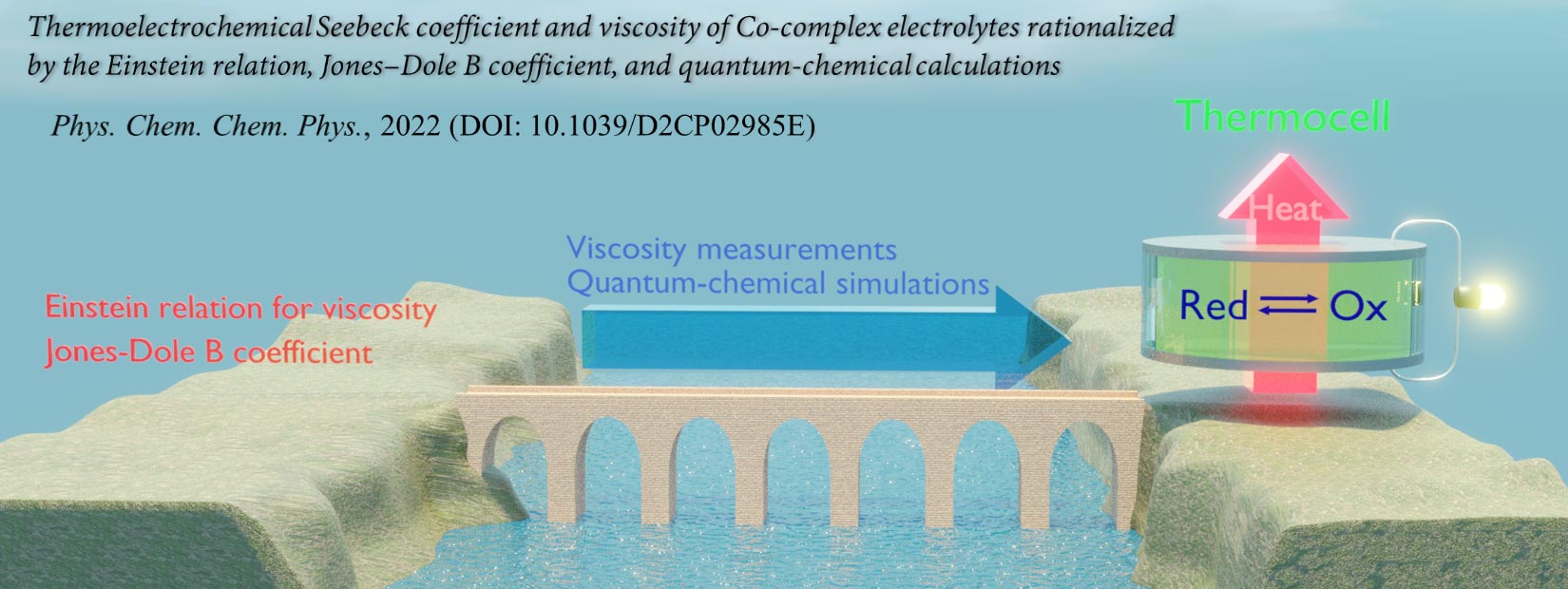
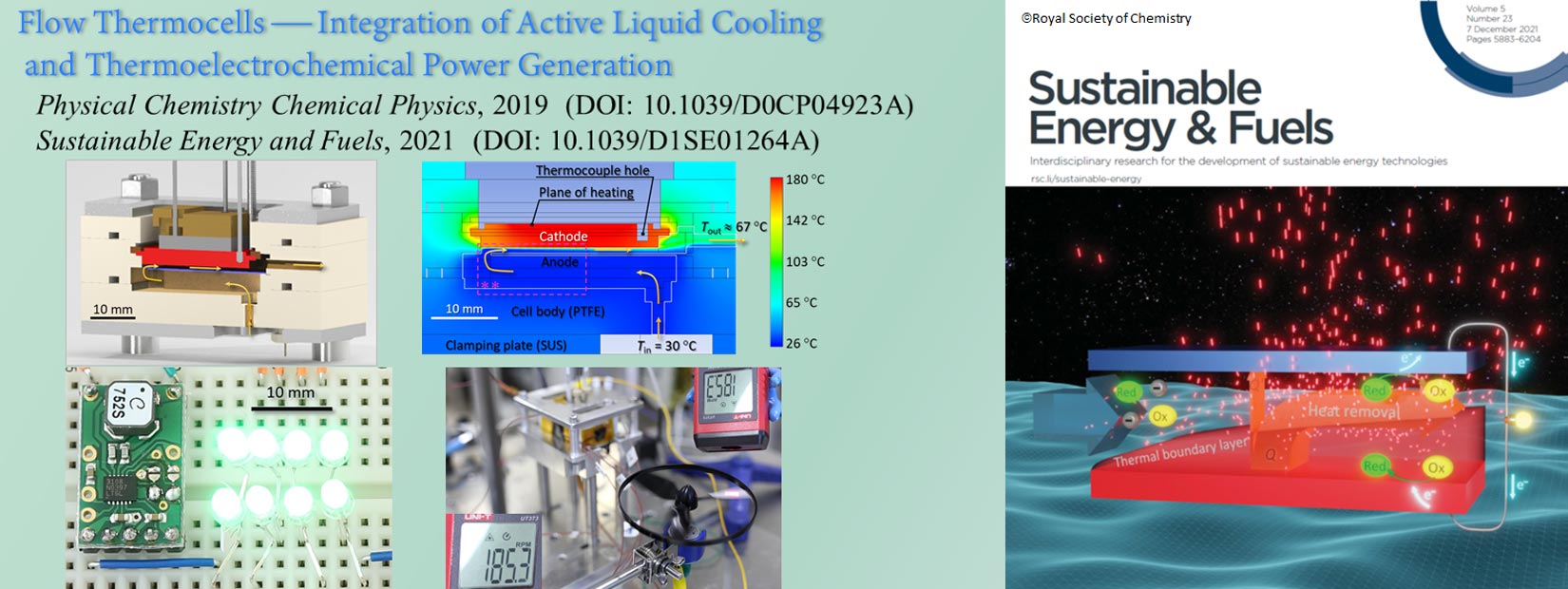
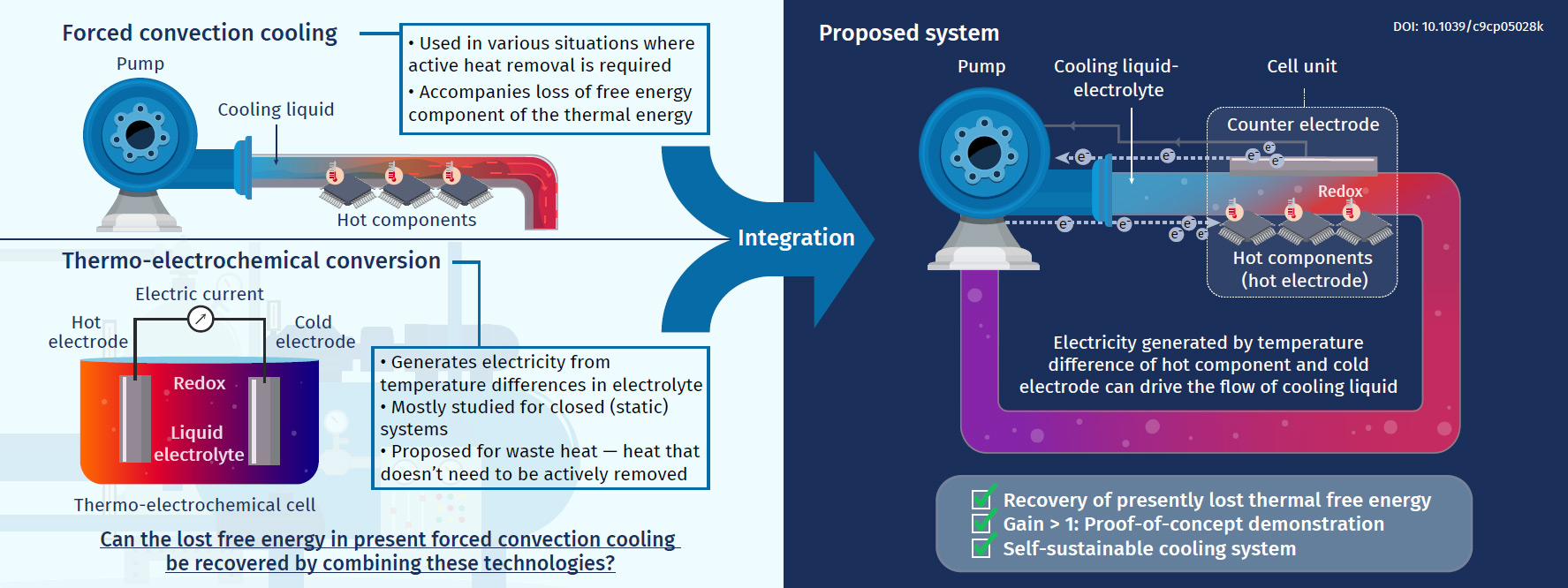
Photon upconversion to increase solar energy utilization efficiency
Light is an electromagnetic wave, as shown by the fact that monochromatic light obtained using a glass prism has a unique wavelength. Light also behaves as a collection of elementary particles called photons whose existence is manifested by the photoelectric effect. Light energy (photoenergy) is especially important among many possible forms of energy. Sunlight is the only energy supplied to the earth from space. In addition, light has many important practical functions to convert energy and matter to more useful forms. For example, using photovoltaics, photoenergy can be converted to more useful electrical energy. Using photosynthesis materials or photocatalysts, photoenergy can be converted to various storable forms of chemical energy like sugars, proteins, hydrocarbon fuels, and hydrogen gas.
However, the efficiencies of these photoenergy conversion systems suffer from a fundamental limitation. This originates from the fact that only photons possessing higher energies than the bandgap energy of semiconductors or the gap energy between the highest occupied and lowest unoccupied molecular orbitals of molecular materials can be used. Photons with lower energy than these intrinsic gap energies of materials are wasted at present regardless of the power of the incident light. Because the energy of a photon is inversely proportional to the wavelength of light, there is a “threshold wavelength” that represents a boundary separating the usable and unusable spectral regions of sunlight. That is, incident light that has a longer wavelength than this threshold wavelength is useless for photoenergy conversion, resulting in the aforementioned limitation of conversion efficiency. For example, the water-splitting reaction to generate hydrogen gas by a photocatalyst can usually be achieved only by light that has a wavelength shorter than blue (∼450 nm). Photosynthesis in plants can only be driven by light whose wavelength is red or shorter. Light that can generate electric power in amorphous silicon photovoltaics is limited to wavelengths shorter than dark red (∼720 nm). Therefore, given the solar spectrum on earth, numerous photoenergy conversion systems display fundamental losses of energy.
Photon upconversion (UC) is a technology that circumvents these fundamental losses and converts presently unused photons to usable ones. Previously, UC was only possible for high-intensity laser light possessing linear polarization. Recently, a method of UC that uses properly designed intermolecular energy transfer between organic molecules has emerged and found to be applicable to low intensity and randomly polarized light including normal sunlight. Thus, this UC method has great versatility with broader application prospects. Realization of such UC with high efficiency would not only increase the efficiencies of aforementioned photoenergy conversions but also broaden the possible applications of light.
We started studies on this UC technology soon after its emergence and have reported several unique achievements. This research field is currently rapidly growing, through which exciting frontiers and questions are emerging. Our present aims are to elucidate such questions and develop novel UC materials suitable for application. To attain these goals, we are conducting highly interdisciplinary research encompassing physical chemistry, mechanical engineering, photochemistry, and materials science.
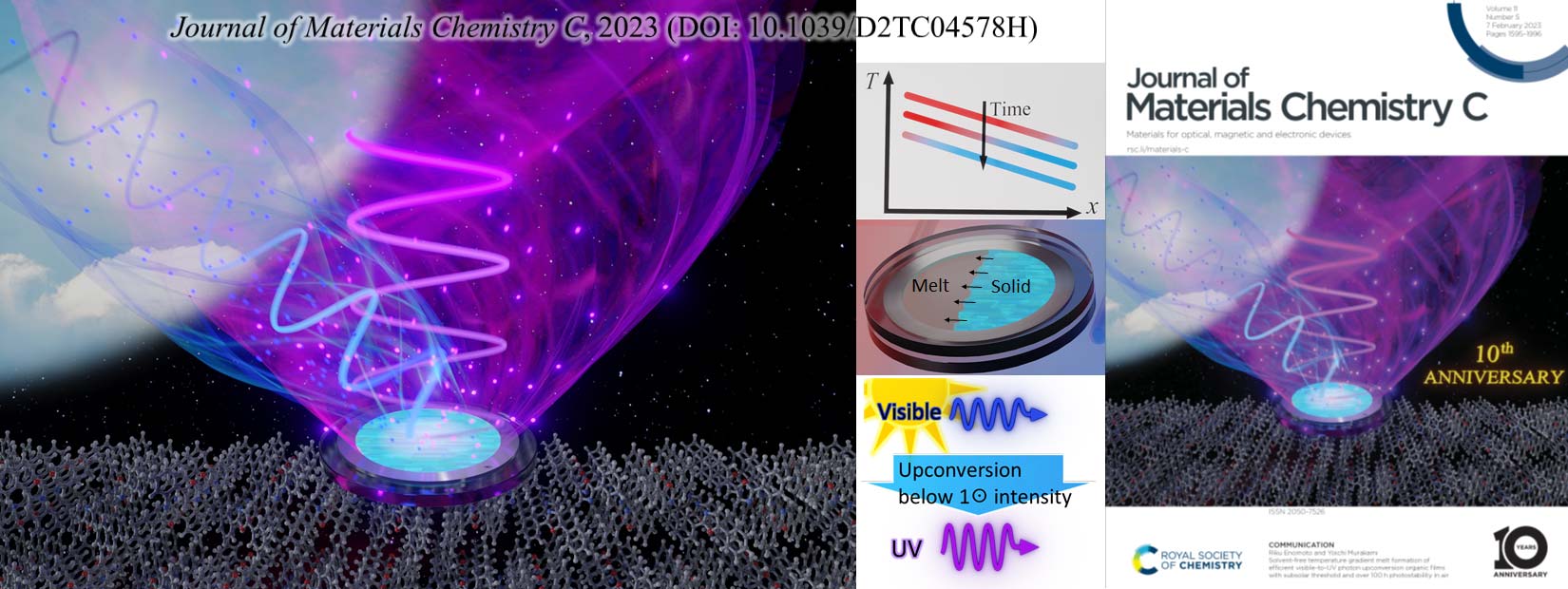
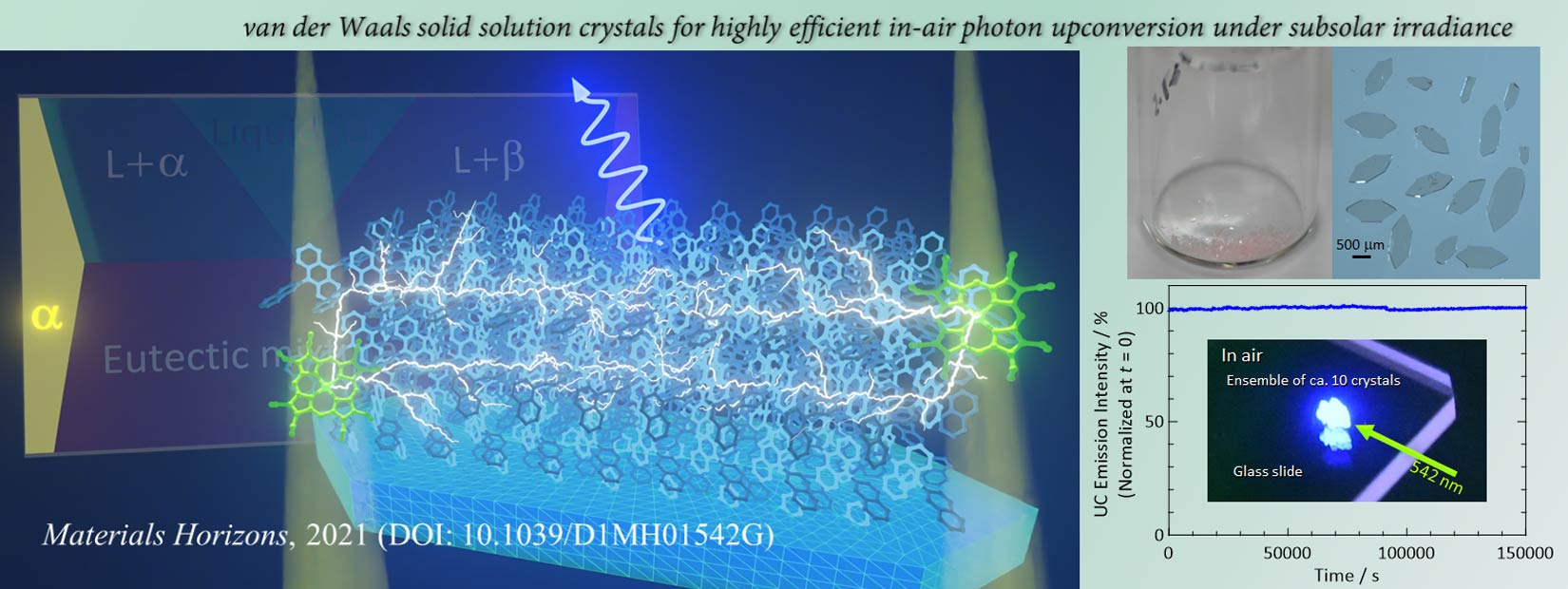
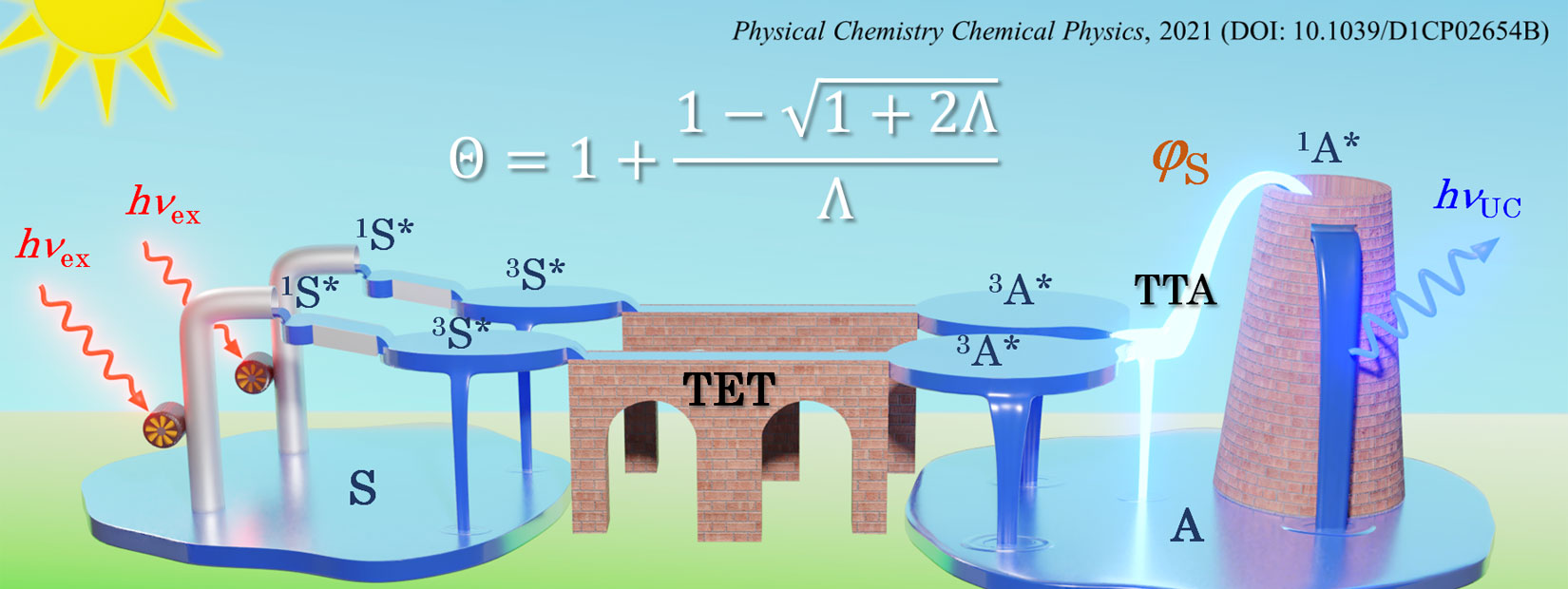